Bringing Astrobiology into focus
Although the question of whether life could exist in other worlds apart from our planet is as old as western culture (it was already raised by ancient Greek philosophers), it could be asserted that only after the advent of the “space age” the matter has reached the status of a scientific concern. Nowadays, astrobiology permeates widely the field of space sciences, and it is the main reference subject in some research areas such as planetary sciences. Furthermore, the astrobiological perspective guides some study lines in different disciplines, from biology and astronomy (obviously), to geology, through chemistry and biochemistry, physics etc., making astrobiology a most typical transdisciplinary area. Therefore, astrobiology is somehow a field in construction, and even its pillars have still to be accurately outlined. This is the case with one of its most basic questions: the definition of life; a cardinal matter in order to determine the further research strategies that have not been appropriately solved yet.
It is a difficult problem since there is only one known example of life, the terrestrial one, which, in addition, is unique. Every single organism on Earth, from bacteria living deep in the crust to whales are nothing but different manifestations of the same kind of life, and all of them (incluiding us humans) are more or less closely related. In this sense, astrobiology is a lacking-in-substance discipline, given that its study subject, different kinds of life from that on Earth, have not yet been found.
The key question: What is life?
To extract a general definition from a particular case is not an easy task and, in addition, it should be outlined not only from a descriptive perspective, but also as a means to comprehend the crux of the phenomenon. That is to say, is not just about characterizing what life looks like, how it acts with relation to its surroundings (the environment), and the effects it causes on it. It is as well necessary to understand what the nature of the process is; what are the physical laws determining its emergence and maintenance, a matter connected directly with the conundrum of the origins of life on Earth. Fortunately, there are interesting approaches, derived from thermodynamics, that have led to the now broadly admitted conviction that life is nothing but a sort of dissipative structure like those identified by Ilya Prigogine. This way, life would be a process that comes to alleviate thermodynamic tensions generated within an energy flux, which keeps systems involved away from equilibrium against their tendency to head towards it as the second law of thermodynamics describes. The further away a system is from its comfortable equilibrium state, the stronger the reaction of the system is to turn back to this state. Under these conditions, Prigogine’s dissipative structures appear as a shortcut to reach the equilibrium.
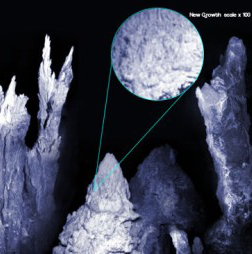
structures in which ultimately are located the primordial events
that gave rise to the emergence of life.
Credit: National Science Foundation/University of Washington
In this new scenario, the ingredients of the old primordial soup proposed by Urey and Miller as the engine of life’s birth were relegated to the role of supporting actors, and the focus has shifted to the conditions themselves, as well as on the particular tension that could have driven the start of the dissipative process we recognize as life. Some audacious scientists, notably Michael Russell, Willliam Martin and Nick Lane (among others of course), have advanced along this line contributing some innovative ideas. In the first place, these authors have pointed to the relief of a particular tension or disequilibrium as the essence of life: that produced between carbon dioxide (CO2) and Hydrogen (H). Furthermore, they have put life’s starting point on the ocean floor, in the so-called “white” hydrothermal vents (in contrast with the “black” ones firstly proposed by Günter Wächtershäuser, pioneer of the “metabolism first” theory regarding the origins of life). They are geological constructions built up by the upward flow of hot water across the crust and into the ocean, with the materials from the mantle it carries. Their inner structure shows tiny cell-like compartments in which chemical conditions are slightly different with respect to the exterior (the water flow itself), allowing the occurrence of the core processes of life. These vents offer the suitable conditions for these species to react, giving place to the dissipative cycle of reactions that define the functional basis of life.
Hence, regarding the core events in the origins of life, organics are not the key; they have been found to be widely distributed in very different celestial bodies all over the universe, including comets and cosmic dust so, in this sense, their presence can be considered as trivial. Neither are “informational” molecules, ARN in the first place, that have been also proposed as the nucleus of the life’s emergence; it is difficult (nearly impossible), to figure out a physically coherent way through which ARN were to agglutinate around itself the huge, powerful machinery that a cell (the basic unit of life) consists of. Both, although they are as well essential elements of life as we know it, came in a second stage to reinforce a process started around the funnelling of an energy flux that kept this particular geochemical system away from its equilibrium state. Life would have appeared as a means to relax the tensions and to eliminate more efficiently the gradients caused by the said flux. As Eric Schneider asserted, “nature abhors a gradient”, a fact which results from the validity of the second law of thermodynamics. In his last book, The vital question: why is life the way it is?, Nick Lane has even ventured that perhaps the scenario, the actors, and the sequence of events that led to the emergence of life on Earth are universal, and it can only take place under these particular circumstances, that is to say, in environments in which H and CO2 meet each other, within a womb of liquid water, an energy flux, and the physical structures that determine suitable conditions for the reaction between those two species to proceed.
Hence, regarding the core events in the origins of life, organics are not the key; they have been found to be widely distributed in very different celestial bodies all over the universe, including comets and cosmic dust so, in this sense, their presence can be considered as trivial. Neither are “informational” molecules, ARN in the first place, that have been also proposed as the nucleus of the life’s emergence; it is difficult (nearly impossible), to figure out a physically coherent way through which ARN were to agglutinate around itself the huge, powerful machinery that a cell (the basic unit of life) consists of. Both, although they are as well essential elements of life as we know it, came in a second stage to reinforce a process started around the funnelling of an energy flux that kept this particular geochemical system away from its equilibrium state. Life would have appeared as a means to relax the tensions and to eliminate more efficiently the gradients caused by the said flux. As Eric Schneider asserted, “nature abhors a gradient”, a fact which results from the validity of the second law of thermodynamics. In his last book, The vital question: why is life the way it is?, Nick Lane has even ventured that perhaps the scenario, the actors, and the sequence of events that led to the emergence of life on Earth are universal, and it can only take place under these particular circumstances, that is to say, in environments in which H and CO2 meet each other, within a womb of liquid water, an energy flux, and the physical structures that determine suitable conditions for the reaction between those two species to proceed.
Life anywhere else
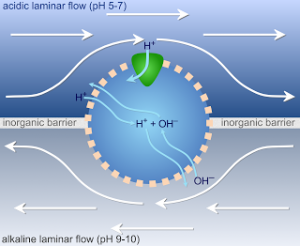
the inner structure of the white hydrothermal vents and the
basic chemical processes in life origins.
These new ideas provide a valuable reference when choosing priority targets in which it should be preferential to perform the search for extraterrestrial life. Although it could be thought that they constraint the possibilities of finding it by reducing the number of likely inhabited worlds to the population of carbonaceous rocky bodies containing liquid water, the fact is that just in the Solar System there are a number of objects that, presumably, accomplish the basic requirements: Europa or Enceladus, moons of Jupiter and Saturn respectively, are provided with huge subsurface oceans in contact with a rocky core heated by tidal friction, from which energy flows outward and into the overlying water, offering (perhaps) the chance for life to flourish according to this conception. On the other hand, Mars, as is known after several decades of exploration, was very similar to the Earth at its beginnings, and life could have arisen there the same way it did here. If that was the case, life there could have remained thriving in the same places that once were its cradle, provided the availability of liquid water. After the last discovery of transient liquid water on present day Mars surface, the permanence of deeper reservoirs in the crust must be determined, and it cannot be asserted conclusively right now that Mars is an inert planet. Indeed, Mars will probably be the first place in which life will be searched for in a direct and active way, as can be confirmed by taking a look at its exploration schedule, which shows the strength of astrobiology as a promising field of research within space sciences.
Beyond our Solar System, astrobiological investigations depend on the use of remote sensing instruments, so the research work in this sense is aimed to outline procedures and methods of detecting traces that could be unambiguously identified as signs of life. There are two main lines in this area: the direct observation of some features of a biosphere, such as the presence of photosynthetic life on the surface of a planet, and the detection of chemical disequilibrium in the atmosphere that could be explained as a result of biological activity. In the first case, some constraining conclusions are derived from evolutionary biology. Simple (bacterial-like) life and complex (multicellular) life are two different matters, as can be inferred from an analysis of life history on Earth. Whereas simple life could be considered a necessary process that starts every time the conditions are appropriate, complex life could be a result of particular contingences that just happened once on Earth, i.e. those that gave place to the advent of the eukaryotic cell, an event that is considered as a mere chance (this matter would deserve a post apart). Thus, since surface life seems to be a prerogative of biological complexity, maybe it could be expected to be rare, unlike simple life which is thought to be widespread all over the universe. In this case, the features that are supposed to be present in life wherever it arises are helpful, because they provide precise biochemical pathways with particular by-products, some of which are eliminated as gaseous waste that can be tracked in the atmospheres.
In this context, atmospheric science appears as a core discipline regarding astrobiological research. Even in the case of Mars, the study of its atmosphere will be crucial in order to unveil, for example, one of the most disturbing discoveries of Curiosity: the unexplained fluctuation of the atmospheric methane concentration registered through the analysis performed during the mission. Almost all the methane present in Earth’s atmosphere is a by-product of biological activity, and just a small fraction of the total amount comes from volcanic activity. Since Mars is a planet without volcanism nowadays, the matter of the methane poses a possible biological dimension that must be investigated. Atmospheric sciences, once more, can provide, in a first stage, models to discard photochemical processes happened in the atmosphere or in the interface ground/atmosphere that could account for the methane presence (and for its sudden and unexpected disappearance, another disturbing conundrum of the issue).
Beyond our Solar System, astrobiological investigations depend on the use of remote sensing instruments, so the research work in this sense is aimed to outline procedures and methods of detecting traces that could be unambiguously identified as signs of life. There are two main lines in this area: the direct observation of some features of a biosphere, such as the presence of photosynthetic life on the surface of a planet, and the detection of chemical disequilibrium in the atmosphere that could be explained as a result of biological activity. In the first case, some constraining conclusions are derived from evolutionary biology. Simple (bacterial-like) life and complex (multicellular) life are two different matters, as can be inferred from an analysis of life history on Earth. Whereas simple life could be considered a necessary process that starts every time the conditions are appropriate, complex life could be a result of particular contingences that just happened once on Earth, i.e. those that gave place to the advent of the eukaryotic cell, an event that is considered as a mere chance (this matter would deserve a post apart). Thus, since surface life seems to be a prerogative of biological complexity, maybe it could be expected to be rare, unlike simple life which is thought to be widespread all over the universe. In this case, the features that are supposed to be present in life wherever it arises are helpful, because they provide precise biochemical pathways with particular by-products, some of which are eliminated as gaseous waste that can be tracked in the atmospheres.
In this context, atmospheric science appears as a core discipline regarding astrobiological research. Even in the case of Mars, the study of its atmosphere will be crucial in order to unveil, for example, one of the most disturbing discoveries of Curiosity: the unexplained fluctuation of the atmospheric methane concentration registered through the analysis performed during the mission. Almost all the methane present in Earth’s atmosphere is a by-product of biological activity, and just a small fraction of the total amount comes from volcanic activity. Since Mars is a planet without volcanism nowadays, the matter of the methane poses a possible biological dimension that must be investigated. Atmospheric sciences, once more, can provide, in a first stage, models to discard photochemical processes happened in the atmosphere or in the interface ground/atmosphere that could account for the methane presence (and for its sudden and unexpected disappearance, another disturbing conundrum of the issue).
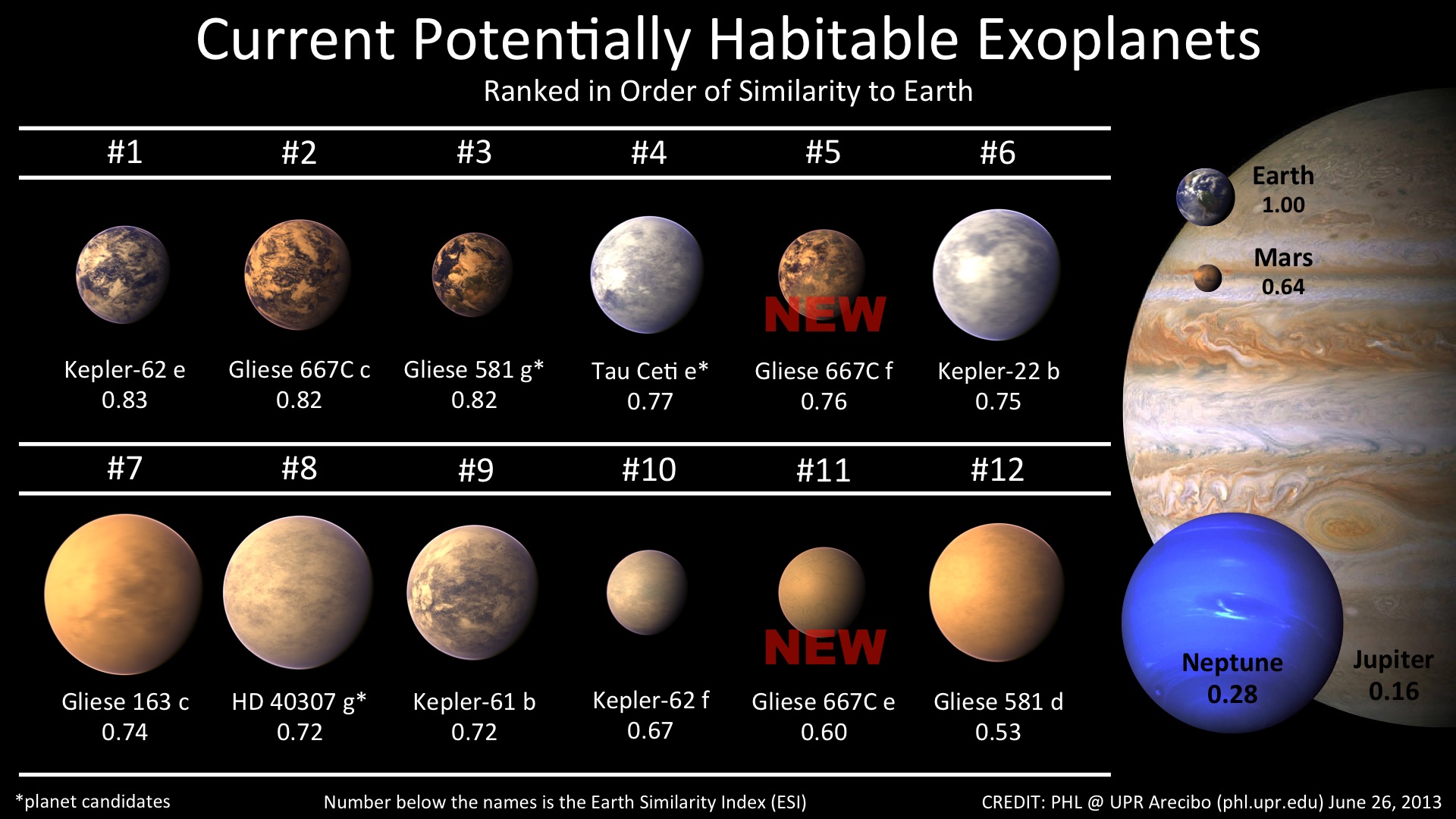
In our cosmic neighbourhood, the search can be performed in a direct way by means of robotic probes, as indeed is being already done step by step, more than all on Mars, the main target of astrobiological research currently. Jupiter’s moon Europe could be the next destination with respect to astrobiology, and then Titan and Enceladus will probably follow.
Beyond the Solar System, the census of planets is increasing rapidly, and the search in them relies mainly in the capability of the atmospheric studies to implement analytical methods to detect conclusively signs of life in their atmospheres, a field that, as astrobiology itself, is quite active at the moment.
References
Michael Russell, editor, Abiogenesis and the origins of life, NASA, Planetary Science and LifeDetection Section, Jet Propulsion Laboratories, California Institute of Technology, Pasadena.
Nick Lane, The Vital Question: Why is life the way it is?, Profile books, London 2015, ISBN 978 1 84765 880 7.
Nick Lane, The Vital Question: Why is life the way it is?, Profile books, London 2015, ISBN 978 1 84765 880 7.
Comentarios
Publicar un comentario